Tidal disruption events around black holes are rare. Predicting them is like predicting Earthquakes; you might know roughly where to look, but you have no idea when one will happen. In a Q&A with Aether, Dr Charlotte Angus of the Niels Bohr Institute at the University of Copenhagen outlines the science behind a TDE that occurred in 2020.
DOI.ORG/10.58214/CANBI0703

Profile: Dr Charlotte Angus
Dr Charlotte Angus is a Research Fellow at the Niels Bohr Institute at the University of Copenhagen, working primarily on supernovae and exotic extragalactic transients.
She is heavily involved in several optical transient surveys, including the Young Supernovae Experiment.
In 2020 an intermediate-mass black hole lurking undetected in a dwarf galaxy, revealed itself to astronomers when it gobbled up an unlucky star that strayed too close. The shredding of the star, known as a ‘tidal disruption event’ or TDE and named AT2020neh, produced a flare of radiation that briefly outshone the combined stellar light of the host dwarf galaxy, and could help scientists better understand the relationships between black holes and galaxies.
The flare was captured by astronomers with the Young Supernova Experiment (YSE), a survey designed to detect cosmic explosions and transient astrophysical events. An international team led by scientists at UC Santa Cruz, the Niels Bohr Institute at the University of Copenhagen, and Washington State University were behind the discovery.
Aether caught up with first author Dr Charlotte Angus, of the Niels Bohr Institute at the University of Copenhagen, to discuss the discovery and what it means for the future of black hole astrophysics.
Aether: How common are tidal disruption events and how exactly do you capture them?
CA: They’re quite rare. There aren’t that many TDEs that I know about, and we have only really known about them in the last 15 or so years. The sample size is still in the few hundreds, so there aren’t that many of them compared to other transient events such as supernovae or stellar flares. Those number in the thousands whereas TDEs are about a thousand times rarer than a star exploding in the same volume of universe.
Which means capturing them is difficult. But we believe these events typically, although in theory they don’t necessarily have to, occur within the hearts of galaxies. That is where you would expect a black hole, or a supermassive black hole to be within a galaxy; roughly within the centre, which helps us to identify them.
If there is something that is rising in brightness very quickly at the centre of a galaxy, that suggests that perhaps this could be related to something happening with the black hole. The event rises in brightness over a period of time, peaks, and then starts to slowly decline.
The majority of TDEs are found after they have reached their brightest point because we normally look for particular behaviours after this point. They do not typically change a lot in terms of their colour; it’s a reflection of the temperature of the event, and they are quite slowly evolving. They also tend to decline at a fairly particular rate, which is indicative of the accretion of material onto the black hole that’s creating it.
Once we have identified an event which fits these behaviours, we obtain a spectrum to confirm it is a TDE. This is kind of what makes AT2020neh a little bit unique, in that we identify this event well before it started peaking and got an early classification, which means that we have a very good series of follow-up observations of this event.
We would like to try and find more of these events earlier, identifying them as they rise, but that is difficult because often they get confused with supernovae that happen to be quite close to the centre of the galaxy as well.
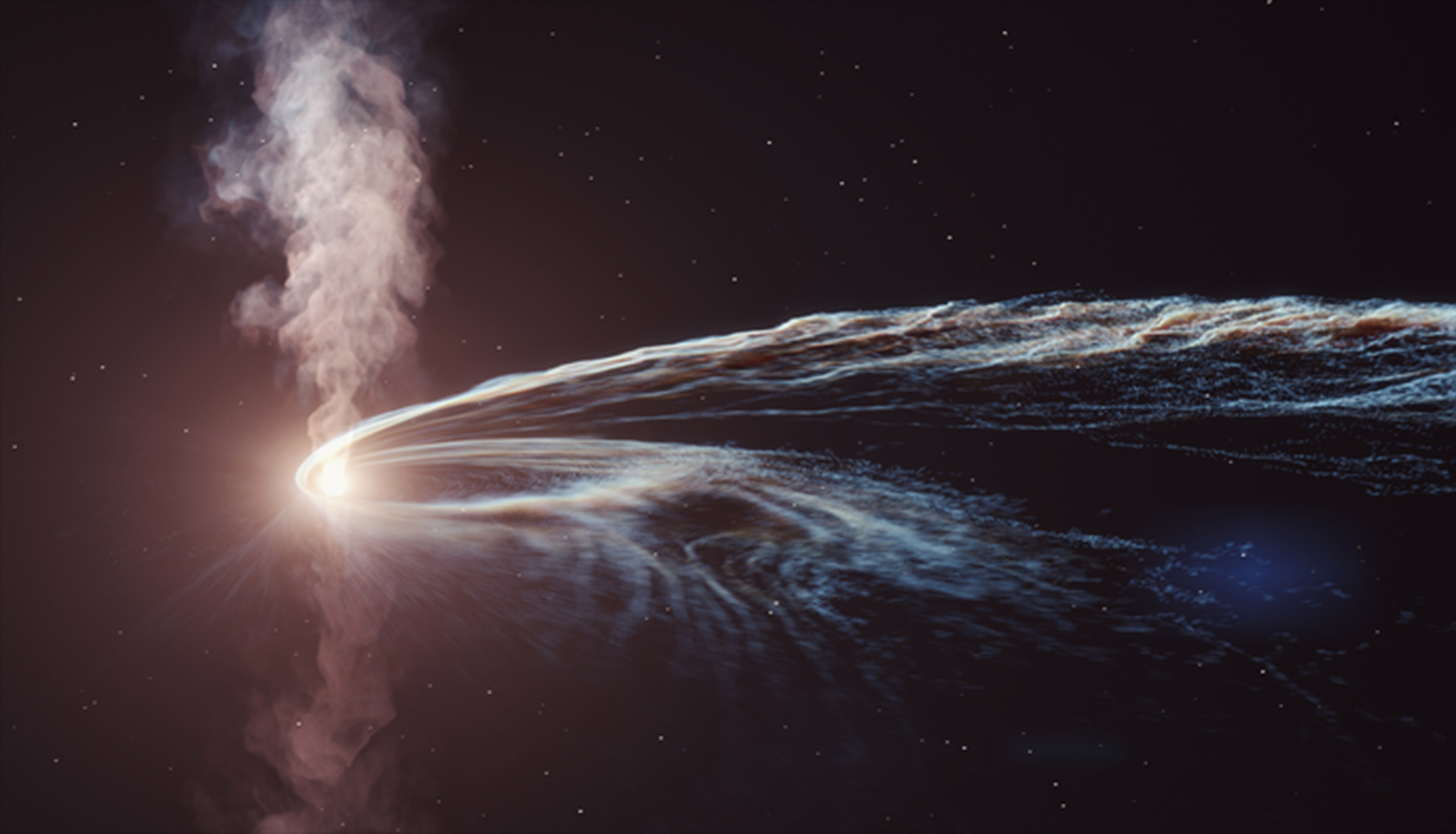
Artist’s illustration of tidal disruption event AT2019dsg where a supermassive black hole spaghettifies and gobbles down a star. Some of the material is not consumed by the black hole and is flung back out into space. © DESY, Science Communication Lab.
Aether: A supernova is the death of a star and it’s explosive, but are TDEs a live star or a dead star that is being shredded in a black hole?
CA: Most of the time it is probably a live star. There’s no reason why you couldn’t have supernova remnants of a dead star passing too close to the super massive black hole, but I think the probability of that happening is really small. We assume these are live stars that get too close to a black hole and get caught within its gravitational influence.
Aether: How can you measure the mass of black holes through tidal disruption events when they are as rare and unusual as they are?
CA: The theory behind this is that the rise time of the tidal disruption event, so the time it takes to reach peak luminosity, is related to the rate at which material will fall into the central back hole. And that rate of falling in, is directly related to the mass of the black hole.
If you have a not very big black hole, you would expect a rising time that is much shorter than if you have a bigger black hole. What we do is take theoptical imaging of the event and use these data to model its full evolution. Our model determines from this information exactly how long it took the TDE to rise, and from this predicts the mass range of the black hole, which would have produced this event.
In our case, we were lucky because we have very constraining data from our combination of Young Supernova Experiment plus the Zwicky Transient Facility survey. Because the observations from the two surveys are quite close together, we have a very good constraint on when the event started. That means that we can model quite well exactly how long it takes the TDE to rise, and therefore constrain the mass of black hole fairly well for this particular methodology.
We also cross-check this. There are a few known relationships between the mass of a black hole in a galaxy, and some of the galaxy’s properties. For example, the total mass of the stars within the galaxy is related to the mass of the black hole and the velocities at which those stars are moving around the galaxy, is related to the mass of the black hole as well.
There is also a process called spectral energy distribution fitting, which is looking at how bright the galaxy is at different colours, fitting lots of templates to those colours, from which you can infer the population of stars, which is producing those colours, and therefore the mass of the stars in the galaxy.
We used that information to infer the mass of the black hole, which we found to agree with our tidal disruption event model.
Using a very high-resolution spectrum we can look at motions of stars in the galaxy and determine their velocity. Putting that additional information into the known relationship also suggests that we have this intermediate-mass back hole sitting at the centre.

The irregular dwarf galaxy, NGC 1156, is located around 25 million light-years from Earth, in the constellation Aries © Public domain.
Aether: Are you able to predict these events or is it simply a case of spotting them?
CA: It’s very much a case of spotting them, but we do know that they are more likely to occur in certain environments. In order to get a tidal disruption event, you need a star to go near a black hole, otherwise, it simply won’t happen.
We know the kinds of galaxies they prefer, and therefore, in theory, if you have like an infinite amount of time, you could watch these galaxies and eventually, you’re likely to observe one in one of these galaxies. These galaxies are generally what we call ‘centrally concentrated’. It means you have got lots of stars close to the centre of a galaxy, and if you have lots of stars then it is more likely one will happen to stray too close to the black hole.
galaxy systems because stars are moving around during the disruption, which is pushing more stars closer to the black hole. We know that they’re more likely to occur there, but again we can’t predict them. We just have to keep watching the night sky and waiting.
Aether: How has this specific event furthered our knowledge of black holes?
CA: It is one of a few intermediate-mass black holes in a dwarf galaxy, which has been confirmed without the presence of what we call ‘existing accretion’ within the galaxy.
We see black holes because they swallow things. Some galaxies in the universe have central black holes that receive constant streams of material that fall into them, which causes the black hole to radiate light at different wavelengths. These are what we call ‘active galactic nuclei’ and these have been used to infer the presence of intermediate-mass black holes in some other galaxies. But not all black holes and galaxies are constantly accreting.
The kind of short accretion events that are produced by a tidal disruption event are a nice window into looking at these non-active black holes. Therefore, we can use them to explore the population of black holes that are not always actively accreting.
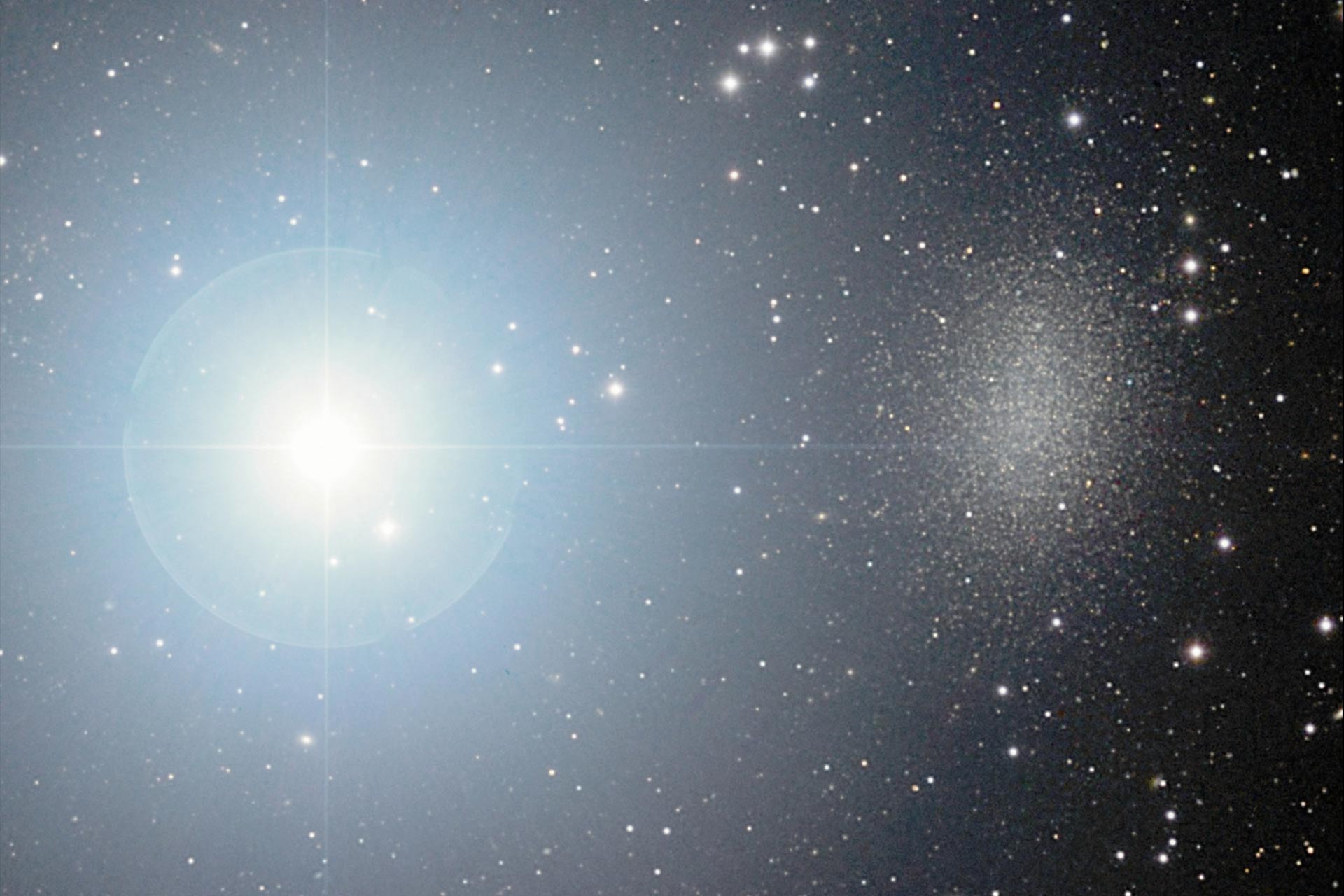
Regulus and the dwarf galaxy Leo I. © ESO/ Dss2/ Giuseppe Donatiello.
Aether: How can your research assist in working out how supermassive black holes develop?
CA: The big question in black hole astrophysics is we have no idea how these really massive ones, like the one at the heart of our galaxy, grow. We believe that they grow from what we call ‘seeds’, and these seeds are black holes with the same masses of an intermediate-mass black hole. Those seeds grow through acquiring more material over time, but we don’t know how those seeds form in the first place.
There are three competing mechanisms for how we think these seed black holes form.
The first is that there is a massive cloud of gas, which directly collapses into a black hole in one go. This black hole would be of intermediate mass.
A second mechanism is that there were incredibly massive stars in the early universe, and when they reach their supernova endpoints, their cores are so massive that they cannot really, like most core-collapsed supernova, produce a neutron star from their core. In this scenario, the density of the core is so great that the neutron star cannot support itself, so it collapses further to form a black hole. Because it is forming from an exceptionally massive core and an exceptionally massive star, it ends up as an exceptionally massive black hole.
The third mechanism is that there is a build-up of smaller black holes. This happens in areas where dense clusters of stars, which are not quite so massive, have lots of dynamical interaction between them. Because of this dynamical interaction, eventually, you get to a point where there are black holes that interact with each other and merge. It is a bit like the first gravitational wave event we detected, where two smallish black holes merged to form a bigger black hole.
Over time these build up to form these intermediate-mass black hole seeds. It is a very difficult field. Theoretically, all of these models can produce this seed population of black holes that we need to grow into our current supermassive black holes, but determining which is the predominant mechanism is really difficult.
What we can do is study intermediate-mass black holes in effect in reverse because it’s predicted that these different formation mechanisms will influence the shape of the relationship between the mass of the black hole and the mass of the galaxy influences at the low mass end. Basically, the slope of the correlation is altered at the low mass end. If we can find more of these, then we can determine whether there is one seeding mechanism, which is preferred over another.
The unique thing about AT2020neh is that it gives you this insight into this quiet, black hole population within these within these small galaxies. If we can find more of these in future surveys, then this can provide us with information as to how these more massive black holes are being seeded and then grow.
